Some magnetic materials are able to absorb electro-magnetic waves and transform them into heat. But how do they do it? And is there a single mechanism or an optimum frequency for this process? To answer these and other fundamental questions of magnetic heating, first some introductory concepts must be clarified related to the magnetic response of the materials to a magnetic field.
Ferromagnetic materials, like iron, are materials that remain magnetized when the magnetic field is removed. We experience this effect in our daily life, for example when the fridge magnet that you brought from your last holidays still sticks to the fridge without the need of an external magnetic field. This property is known as magnetic remanence. On the contrary, paramagnetic materials lose their magnetization completely when the magnetic field is removed with no remanence and consequently they do not stick to the fridge. In the first case, the materials are able to absorb magnetic energy and transform it into heat; in the second, they lose completely the magnetic energy when the field disappears without heat dissipation.
Magnetic materials at nanoscale
Ferromagnetic materials modify their magnetic response when the size is reduced to nanoscale. At this small sizes, the remanence of static magnetization curves decreases to zero and reproduce a S-shape like the one presented inside the cloud of Figure 1. This magnetic response receives the name of “superparamagnetic” because it presents some paramagnetic features like the lack of remanence but reaches its maximum magnetization at relatively low fields. According to the criteria mentioned before, at static conditions, the superparamagnetic nanoparticles do not dissipate heat. However, superparamagnetic nanoparticles recover their usual ferromagnetic response when alternating magnetic fields are applied (dynamic range). The relaxation phenomena responsible of the lack of remanence in static regime disappear when the frequency of the field applied is high enough.
A tool for remote control
Thus, superparamagnetic nanoparticles are interesting agents for contactless heating. In the absence of a magnetic field, they will behave as non-magnetic (zero remanence) avoiding problems of aggregation and precipitation. Thus, they can be dispersed in liquid media or conjugated with other molecules like enzymes. In the presence of high frequency magnetic fields, they turn into local nanoheaters creating hot spots near the surface of the nanoparticles while the rest of the liquid remains cold.
Understanding the process at atomic scale
There is still an open question. Why ferromagnetic materials lose their remanence at the nanoscales? To answer this question, we must investigate the magnetic materials at the atomic scale. The ferromagnetic materials are made of atoms that possess small compasses known as magnetic moments. These magnetic moments align with the magnetic field applied and are responsible for the magnetization of the material. In bulk materials, these moments are fixed in a common orientation but in nanoparticles this orientation can change due to thermal agitation. The magnetic moments are aligned when the magnetic field is applied, but when it is removed, they can rotate in a process known as relaxation. The rotation of the moments can take place by two routes. In Néel relaxation, the nanoparticle is fixed and the magnetic moments rotates respect to the atomic positions. Whereas in Brownian relaxation, the nanoparticle rotates as a whole.
The relaxation is the key parameter to explain the dual behaviour of ferromagnetic nanoparticles. If relaxation is faster than the oscillation of the field, the magnetic moments results misaligned at zero field and the remanence of the system is vanished, what corresponds to superparamagnetic regime. When the relaxation is slower than the oscillation of the field, the system preserves certain orientation at zero field, what corresponds to ferromagnetic regime.
The optimum set of magnetic field?
The amount of heat generated by the superparamagnetic nanoparticles depends on the frequency and strength of the magnetic field applied. Depending on their size, shape and composition, there is an optimum set of magnetic field conditions to extract the maximum amount of heat from the nanoparticles. In the HOTZYMES project, we are exploring iron oxide nanoparticles with different sizes and shapes to generate a toolbox of magnetic nanoheaters, able to regulate the enzymatic activity by controlling their local temperature (more information in www.hotzymes.eu).
Picture credits: Background photo created by GarryKillian – www.freepik.com
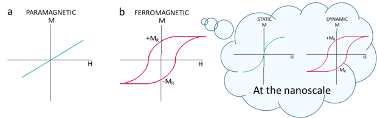